  |
 |
 |
|
Cruising Inside Cells
Dr. Atsushi Miyawaki |
Head, Laboratory for Cell Function Dynamics |
|
 |
The behavior of biochemical molecules moving around in cells makes me think of a school of whales wandering in the ocean, captured by the Argus system on the artificial satellite. When bringing a whale back into the sea - with a transmitter on its dorsal fin, every staff member hopes that it will return safely to a school of its species. A transmitter is now minute in size, but it was not this way before. There used to be some concern that a whale fitted with a transmitter could be given the cold shoulder and thus ostracized by other whales for "wearing something annoying". How is whales" wandering related to the tide or a shoal of small fish? What kind of interaction is there among different species of whales? We human beings have attempted to fully understand this fellow creature in the sea both during and since the age of whale fishing.
In a cell imaging experiment, a fluorescent probe replaces a transmitter. We label a fluorescent probe on a specific region of a biological molecule and bring it back into a cell. We can then visualize how the biological molecule behaves in response to external stimulation. Since fluorescence is a physical phenomenon, we can extract various kinds of information by making full use of its characteristics. For example, the excited energy of a fluorescent molecule donor (energy donor) transfers to an acceptor (energy receptor) relative to the distance and orientation between the donor and acceptor. This phenomenon (fluorescence resonance energy transfer) can be used to identify interaction between biological molecules or structural change in biological molecules. Besides, we can apply all other characteristics of fluorescence, such as polarization, quenching, photobleaching and photoisomerization, in experimentation.
Specific external stimuli cause specific cascade reactions inside cells, so that cell phenomena like differentiation, migration and division will result. Many molecules involved in such an intracellular signal transduction have been identified along with their hierarchical relations. It is not uncommon for us to see a signal transduction diagram in which arrows are used to link molecules to show enzyme reactions and intermolecular interactions.
However, to obtain an all-embracing understanding of a signal transduction system, the diagram must contain three axes in space and the time base, because all events are controlled ingeniously in space and time. This scale over time and space is ignored in biochemical approaches in which electrophoresis is applied to a specimen prepared by grinding millions of cells - which means that the resulting understanding is far from satisfactory. There is a need to employ the so-called real-time and single-cell imaging technique, which allows us to observe events in each living cell in real time.
Let's look back at the progress of technologies in measuring intracellular concentration of calcium ion, which is one of intracellular messengers. Thanks to the development of fluorescent indicators for calcium, as well as to techniques for performing imaging and quantitative evaluation of their signals, it has been made known that cellular functions are caused by dynamic changes in calcium concentration over time and space, including calcium oscillations and oscillations.
|
 |
A feedback mechanism from lower to higher hierarchy acts on the molecular network of a signal transduction system. Also, it involves interaction with other transduction systems. As a result, a biological phenomenon may sometimes react unpredictably to a scientist's arbitrary action. For example, even in an experiment to excessively generate or eliminate specific molecular species, it is important to visualize their behavior not only downstream but also in the entire transduction system. We therefore aim at developing an imaging technique extravagant enough to ascertain when, where, and to what extent (i.e. quantitatively) various events take place inside cells. To describe post-genome project, it is important to attempt to increase parameters that allow for simultaneous observation and are designed to describe intracellular signal transduction systems, rather than just setting holism against the theory of element reduction.
To develop an imaging technique is to struggle against artifact. As in the case of a transmitter on a whale, too large a fluorescent probe can possibly alter the intrinsic behavior of biological molecules. The green fluorescent protein (GFP), which is currently being utilized chiefly in the field of cell biology, is a protein comprised of 238 amino acids. GFP is making itself useful because it autonomously emits light without any cofactor. Nonetheless, it is not fair to say GFP is a small probe. It is necessary to give more careful consideration to the artifact that results from labelling with GFP.
In addition, assume enzymes that are activated are subject to a structural change caused by particular ligands. Suppose that we convert a structural change into a signal change of fluorescence to generate fluorescent indicators for those ligands. The introduction of excessive indicators into cells can cause problems, although this applies in whatever case. First of all, it bears a threat of excessive increase in the enzymatic reactions. On the other hand, if we override the enzymatic activity alone while retaining the ligand-dependent structural change of indicators, ligands will be defeated by indicators - that is, buffering will result.
In this manner, varying degrees of cells will be perturbed as long as an extrinsic fluorescent probe is introduced. As in the case of Mach's sensationalism, there is a need to recognize that it is impossible to get a perfect picture of things. With this determination, we should make our best efforts to gain a better understanding.
|
 |
magnified scene by clicking image
Fig. 1 | Cameleons, or fluorescent calcium indicators developed from GFP and calmodulin. The relative position between the donor and acceptor changes in a calcium dependent manner, so does the fluorescence resonance energy transfer from donor to acceptor. |
|
 |
magnified scene by clicking image
Fig. 2 | Time-lapse change of cytoplasmic calcium concentration in HeLa cells, observed with cameleons. External information (histamine stimulation) is encoded as amplitude and frequency of calcium oscillations. |
|
magnified scene by clicking image
Fig. 3 | Spatial change of cytoplasmic calcium concentration in HeLa cells, observed with cameleon. It matters in which areas calcium concentrations first rise and how they diffuse. |
|
Asked what is the final goal of my research activities, I consciously avoid giving concrete answers like synaptic formation and plasticity, neuronal development and differentiation, and aging-related such and such. We are indeed interested in developing some techniques that are designed to verify hypotheses as to certain biological phenomena of central nerve systems. But for our laboratory that has only recently opened, we should, we believe, be as exclusive and flexible as possible, rather than focusing on some specific fields. We aim at pursuing the true nature of something flexible about cells with a breadth of vision. We attempt to grasp the meaning of a cell which automatically controls the ratio of output (reaction) to input (stimulation).
Cruising inside cells in a supermicro corps, gliding down in a microtubule like a roller coaster, pushing our ways through a jungle of chromatin while hoisting a flag of nuclear localization signal - we are reminded to retain a playful and adventurous perspective at all times. What matters is mobilizing all capabilities of science and giving full play to our imagination. We believe that such serendipitous findings can arise out of such a sportive mind, a frame of mind that prevails when enjoying whale-watching.
|
 |
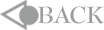 |
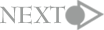 |
|
|