 |
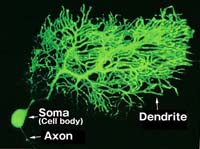
magnified
scene by clicking image
Fig.1 |
Cerebellar
Purkinje cell |
Fig.2 |
Cerebellar
Purkinje cell and its synapse |
|
The organism body is composed
of various types of molecules, such as protein, nucleic acid, lipid, as well as
others. The neural system too, including the brain and the spinal cord, is composed
of these molecules and the so-called higher functions such as memory, learning
and recognition, are actualized by the behavior of these molecules. Therefore,
by understanding the behavior of these molecules, it is possible to elucidate
the mechanisms of the higher functions performed in the central nervous system.
In the central nervous system, neurons make contacts with each another to constitute
a neuronal pathway for information processing. Therefore, the properties of each
neural pathway are determined by the properties of neurons that constituting it.
For example, plastic changes in information processing in the neural network such
as memory and learning occur because of the changes in neuronal properties for
efficient signal transmission. So, what is the molecular mechanism that determines
the properties of information processing in such neurons? So far, various molecules
involved in the brain functions have been identified and their functions as well
have been elucidated, using biochemical, molecular biological methods, and others.
The development of the molecular biological method in recent years has resulted
in better understanding of information processing. It has become clear that cellular
information processing is supported mainly through information exchange among
adjacent molecules and there is a functional subarea (domain) where many kinds
of molecules gather to conduct centralized information processing. Inside cells,
various domains with different molecular structures and functions are formed in
locations, and the exchange of information and molecules among these domains maintain
the structure and function of the entire cell. Particularly in neurons, these
functional domains develop remarkably to enable efficient processing of complicated
information. Fig.1 shows a photograph of a cerebellar Purkinje cell, one of the
largest neurons in the brain. As it shows, neurons are composed of dendrites,
a cell body and an axon. Neurons receive information with there dendrites, and
process it through various compartments in the cell body, and then transmit to
the next cell. As for these dendrites, cell bodies, and axons, though it has been
thought that each of them is a large functional domain, they are actually composed
of smaller domains. Among these domains, the one called "postsynaptic structure"
has been widely noticed in recent years. Neurons form synapses at the dendrites
with axons reaching out from the previous neuron in the neural pathway, in order
to receive information. Inside neurons, information is transmitted in electric
signals based on changes in the membrane potential, while in synapses neural cells
do not connect directly to each other and there is a gap called a"synaptic cleft".
At synaptic cleft, electric signals are temporarily replaced with chemical molecular
signals (neurotransmitters) and transmitted to the postsynaptic cell. Then, peculiar
mechanisms work in the presynaptic structure for the release of the transmitters
and in the postsynaptic structure for the reception of the transmitters respectively.
Fig. 2 is an enlarged area of the Purkinje cell dendrite, in which numerous small
projections can be observed. These projections are called "spines", where the
Purkinje cell receives information from the presynaptic cell. There are 100,000
to 200,000 spines on one cell and the information received here is processed inside
the cell. Inside these spines, a postsynaptic structure is formed to receive information
and transmit it into the cell. Enlarging this part further with an electron microscope,
a distinctive structure can be observed as shown in Fig. 3.
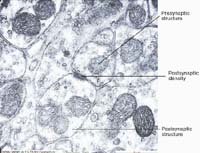
magnified
scene by clicking image
Fig.3 |
Synaptic
microstructure(electron micrograph) |
|
-- |
What
is distinctive in the presynaptic structure is spherical synaptic vesicles, in
which neurotransmitters are accumulated and released to the synaptic cleft according
to the electric signals transmitted through the axon. There is a black high-density
area in the postsynaptic structure, and it is called the"postsynaptic density
(PSD)", the central structure which receives the information of neurotransmitters.
This structure contains receptors which bind to neurotransmitters, enzymes that
control the function of the receptors, signal transduction molecules that transmit
the signals from the receptors into the cell, adhesive molecules that maintain
the synaptic structure, as well as others. It is postulated that there are more
than 30 kinds of molecules gathered within this structure. Though the functions
of each individual molecule in this structure have not been confirmed, the mechanism
of the formation and maintenance of the structure has become clear and well studied
in recent years. There are various kinds of neurotransmitters such as amino acids
and peptides, each of which has its peculiar receptor. As for glutamic acid, one
of the major neurotransmitters of the central nervous system, there are various
kinds of receptors that bind to it and show different reactions upon the binding.
Among these receptors, some show the function of a channel that filters ions and
such receptors are the ones that convert the information of neurotransmitters
directly into electric signals.
|
On the other hand,
receptors that control cellular information processing mechanisms such as the
phosphorylation of proteins do not have the channel function, although they are
receptors for the same neurotransmitters. However, these receptors are related
with one another in the same postsynaptic structure while receiving information
from the presynaptic cell. For example, it has become clear that the property
of inotropic receptors is changed by signals that passed through other receptors.
And such change in the response of receptors to signals due to stimulus from outside
the cell is supposed to create plastic change in signal transmission among neurons
and resulting in the basic process for learning and memory. Therefore, it is necessary
that different types of receptors and molecules that process signals of the receptors
should be gathered and arranged properly in the postsynaptic density. Recently,
the molecules that are involved in the arrangement of these receptors have been
discovered successively and this lead to better understanding of the structure
inside the postsynaptic density.
|
-- |
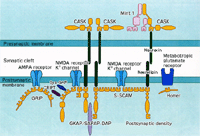
magnified
scene by clicking image
Fig.4 |
Molecular
components around synapse |
|
Fig. 4 is a schema showing the relation between these molecules and receptors
that has so far been made clear. Our laboratory is conducting identification of
the molecules working in synapse formation and information processing in cerebellar
Purkinje cells and granule cells, as well as the analysis of their functions.
Among these molecules, some are supposed to bind with receptors and take part
in their localization, and some others may process dual functions by converting
between function as an adhesion molecule or as a growth factor according to the
signals. The molecules working in such synapses will be identified and the mechanism
for organizing these molecules will also be uncovered in future. As described
above, it is obvious that the cell-interior, which has so far been thought to
be a container full of molecules, is actually having a properly ordered structure
and the functional domains interact with one another to actualize the functions
as a cell. In addition, it is also becoming clear that molecules in these domains
interact with each other to dynamically change their structures and functions.
Such change occurs in neural cells in order to change the properties of cellular
information exchange. This is supposed to be the mechanism in which the higher
functions of the central nervous system such as neural plasticity are based upon.
|
 |
|
|