What a mouse on a virtual track can tell us about how the brain navigates
Virtual reality is an efficient testbed for studying place memory and learning
May 9, 2017
Amanda Alvarez
This post originally appeared on Neurographic
About a dozen years ago, researchers discovered that rodents can navigate in virtual reality (VR). It’s a new twist on the classic mouse maze that allows researchers to precisely measure how far animals travel and control what they see. Importantly for neuroscientists, brain imaging can take place at the same time as animals are performing tasks in VR. Instead of wearing mini-headsets, mice are placed in front of spherical or flat screens and can run on top of a floating trackball.
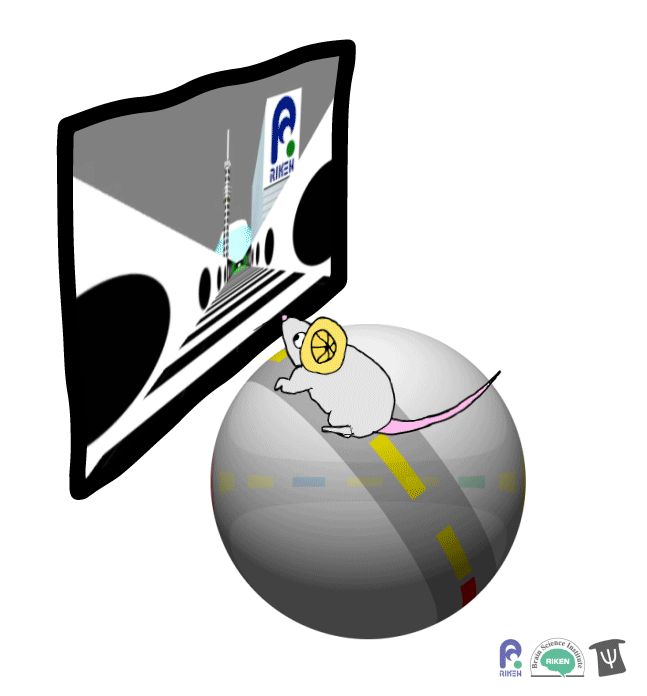
A mouse runs on a trackball to navigate a virtual environment and get a water reward. Image: Adam Phillips/RIKEN
For Masaaki Sato and colleagues, virtual reality opens up a wealth of experiments on navigation, spatial learning and memory in mice. Working in Yasunori Hayashi’s lab at the RIKEN Brain Science Institute, they recently showed that mice need the hippocampus — a brain region that stores memories of places — to remember locations in VR, just like they do in real environments. What’s more, they found that the loss of just one kind of protein in the brain can seriously hinder how well mice learn to navigate in the VR task. Mice that lack the Shank2 protein exhibit impaired spatial learning and mimic a gene mutation found in a small fraction of human autistic patients.
In the experiment, mice ran on a linear track presented on a screen straight ahead. The task was to learn where on the track to stop to receive a reward of a sip of water. Mice were able to learn the location of the reward zone, even when the experiment was changed slightly and the water was moved to a different virtual location or delivered after a delay. To figure out what cues were most useful to the mice in navigation, the researchers changed certain landmarks in the virtual environment, removing a building, tower and mountain that appeared outside the track. Changing these external cues didn’t have much effect on the mice; what really mattered was whether the local cue for the reward zone, a green panel, was present or not.
Aside from vision, mice obviously need other parts of the brain to navigate successfully, even on a simple path. The authors turned off part of the hippocampus using CNQX, a compound that can block certain neurotransmitter receptors and therefore reduce normal brain cell signaling. Mice that got this treatment actually spent more time outside the reward zone, though they could still travel along the track. “The ability of the mice to localize a goal had been abolished by suppressing neural transmission in the hippocampus,” says Sato. A few days later, though, the mice were back to normal and could find the water reward target again. Goal location memories can thus be temporarily inactivated but not permanently lost…that is, unless you’re a mouse missing the Shank2 gene.
The researchers also tested a group of mice lacking this gene, which produces a scaffolding protein that is located at excitatory synapses — connections between neurons— throughout the brain. Mutations in Shank2 have also been implicated in human autism, where some individuals may have impaired spatial learning. In the experiment, Shank2-deficient mice could find the target zone if there was no delay in the water reward, but they never learned to stay put if a delay was introduced; they also had trouble learning a new target zone if it was moved.
“Place recognition memory depends on the hippocampus,” says Sato. “Virtual environments allow us to test how the perception of visual cues interacts with place memories precisely, to determine how each affects behavior.” The particular goal-directed navigation in this experiment relied heavily on the hippocampus, because both a pharmacological block and a genetic deletion affected neurons in this brain area. Sato and colleagues are now pursuing other virtual reality scenarios, including reconstructing the social behavior of mice in response to defined sensory stimuli. “Our goal is to understand how brain circuits work during navigation and learning, and virtual environments let us look at brain activity and behavior simultaneously.”
While virtual reality has taken off as an experimental method in neuroscience, it is not without its drawbacks. There was some concern that mice are not sufficiently visual animals, relying more on whiskers and smell, but Sato says their performance on this and other VR tasks shows that mice do use vision to navigate and form place memories. Another issue arises from head-fixing experimental animals: they may get the same sensory input as in real world navigation, and respond with the same motor actions, but other real-world details — head tilt and acceleration signals from the inner ear, for example — are missing. This may not be especially concerning in experiments like Sato’s that use a linear track, but there is evidence that head or body restraint of animals changes how the brain codes for location. How virtual and real navigation differ in terms of brain activation is itself an engaging research question. Fortunately the very nature of VR that introduces these discrepancies also allows for the experimental fine-tuning and dissociation of sensory and motor information, which is central to modern neural circuit research.
Sato M, Kawano M, Mizuta K, Islam T, Lee MG, Hayashi Y (2017). Hippocampus-dependent goal localization by head-fixed mice in virtual reality. eNeuro, DOI: 10.1523/ENEURO.0369–16.2017