  |
 |
 |
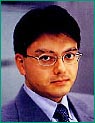 |
Phase II of the Neuronal Function Research
Group
Director, Neuronal Function Research Group |
Dr.
Takao K. Hensch |
|
 |
Much of what we know
about neuronal function stems from the elegant, detailed investigation of single
cells at the biophysical or molecular level. Yet, neurons only realize their full
potential once they are "plugged"
into circuits. During its first phase, the Neuronal Function Research Group at
BSI established a unique focus upon the interface between cellular and systems
neurobiology. This perspective yielded major advances in our understanding of
olfactory (Drs. K. Mori, Y. Yoshihara), cerebellar (Drs. M. Kano, R. Yano), and
visual processing (Dr. T.K. Hensch). Combining classical electrophysiological
and anatomical techniques with modern molecular/genetic approaches has become
the hallmark of our group. In the coming second phase, we hope to explore further
the cellular interactions which endow specific function to networks of neurons.
|
 |
Olfactory
Pathways |
magnified scene by clicking image
Fig.
1 WGA transgene method for selective labeling of neural pathways of interest
By using WGA cDNA as a transgene in combination with a neuron type-specific promotor
element, anatomically-connected and functionally-related neural pathways can be
clearly visualized with great accuracy and high reproducibility. |
Systematic single-unit
recordings, and more recently optical imaging of intrinsic signals1), by Mori
and colleagues have established the functional organization of the olfactory bulb2).
Identification of the 1000 gene repertoire of mammalian odorant receptors has
further made this sensory system accessible to molecular analysis and manipulation.
Sensory neurons expressing a given olfactory receptor converge their axons onto
topographically fixed glomeruli in the target bulb and make synaptic contact with
a specific set of principal neurons, mitral and tufted cells. The overriding mystery
remains how such a precise wiring scheme can be established and maintained. The
Laboratory for Neurobiology of Synapse (Y. Yoshihara, head) will continue to tackle
this question in two ways. First, the ongoing identification of recognition molecules
that may guide individual odorant receptor cell axons to specific glomerular targets
will be expanded. To date, Yoshihara et al. have described several novel cell-adhesion
molecules expressed in robust patterns within olfactory bulb (e.g. OCAM, BIG-1,
telencephalin), as well as the rest of the brain2). Tissue culture and gene targeting
in mice are clarifying their functional role in neurite outgrowth. A complementary
approach using mutant screens in zebrafish will further characterize molecules
underlying olfactory axon pathfinding. Second, an innovative anatomical tract-tracing
technique pioneered by this laboratory aims to elucidate the multiple processing
stages downstream of any given odorant receptor3). Cell type-specific promoters
have been coupled to the gene encoding wheat-germ agglutinin (WGA) to successfully
visualize neuronal circuits emanating from those source neurons in transgenic
mice (Fig. 1). Trans-synaptic transport of WGA expressed in individual odorant
receptor cells is expected to reveal the fine-tuning of connectivity through olfactory
bulb and beyond into cortex during development and plasticity.
|
Visual
Pathways
magnified scene by clicking
image
Fig. 2
Diverse morphology (red, axons; black, dendrites) of inhibitory Inter neuron sub-types
within cerebral cortex. Courtesy of Dr. Y. Kawaguchi (RIKEN & NIPS). |
.Neuronal networks in
the visual system remain obscure despite comparatively many years of study. An
optimal excitatory-inhibitory balance within neocortex appears to determine receptive
field properties such as size, orientation, or direction preference. The Laboratory
for Neuronal Circuit Development (T.K. Hensch, head) has focused upon this changing
balance during early postnatal life. They have demonstrated that the sensitivity
to visual experience first described by Hubel and Wiesel over 40 years ago is
driven by inhibition. Unlike wild-type animals, mice lacking a synaptic isoform
of GABA-synthetic enzyme, GAD65, do not shift their cortical responsiveness in
favor of the open eye when one eye is occluded4). Full ocular dominance plasticity
is restored in an all-or-none fashion when GABAergic transmission is locally enhanced
with benzodiazepines during the brief monocular deprivation. These results indicate
that cortical microcircuits ミ rather than single synapses ミ detect the disparity
between competing sensory inputs to instruct the expression of changes in both
thalamocortical and intracortical connections. Identification of an animal model
exhibiting a robust yet reversible disruption of plasticity in the intact visual
cortex provides a valuable tool for dissecting the mechanisms underlying experience-dependent
changes in the brain. Future work will focus on the rich diversity of inhibitory
interneuron subtypes within neocortex to determine which circuit elements are
especially important for plasticity in vivo (Fig. 2). Moreover, close collaboration
with other institutes at RIKEN, such as the Genomic Sciences Center, promises
to unravel the cascade of events that are triggered when plasticity is switched
on by the appropriate excitatory-inhibitory balance.
|
|
Critical
Periods for Neuronal Circuit Function |
magnified scene by clicking image
Fig. 3
Neuronal circuits in developing visual cortex are shaped by experience during
a limited time in early life. Prematurely raising cortical inhibition (blue arrow)
or genetically maintaining a low level (red arrow) can advance or delay, respectively,
onset of the critical period(yellow) for sensory plasticity. |
Olfaction and vision represent
two extremes with regard to sensory circuit refinement. Throughout life, axons
must repeatedly navigate to their correct glomerular target as receptor cells
turn over in the olfactory epithelium. Instead, right and left eye inputs into
binocular visual cortex compete for space only once in life. What renders one
system continually plastic, while the other is fixed in place? Understanding the
basic mechanism of this dichotomy harbors one of the greatest contributions that
basic neuroscience can make to society: how to appropriately nurture the brain.
The rescue of GAD65 mutants above was possible not only at the peak of the typical
critical period for plasticity, but also later in adulthood5). Conversely, the
onset of plasticity in visual cortex could be accelerated with diazepam in immature
wild type animals. This represents the first example of direct control over the
timing of a critical period, and inhibition holds the key (Fig. 3). Interestingly,
GABAergic granule cells are continuously generated throughout life in the ever
plastic olfactory bulb. In the second phase, we wish to incorporate the exploration
of one other sensory pathway into our group. For example, it would be exciting
to pursue inhibition and critical periods for circuit refinement in the auditory
or somatosensory system. Such whole animal studies would eventually lead us back
to cellular analyses. In particular, how do neurons react when inserted into small
networks of a variable excitatory-inhibitory milieu? Or more specifically, what
is the interaction between diverse types of inhibitory interneuron and what function
(e.g. response synchronization, oscillation) does this impart onto the network
as a whole? Clearly, a fourth team dedicated to the high-resolution study of local
circuit behavior in vitro is also required.
In conclusion, then, we wish to encourage interested candidates in these two areas
to apply for our generously funded positions by the March 15, 2001 deadline. As
rigorously demonstrated for the cerebellum elsewhere at BSI, we now endeavor to
understand how sensory neuron function emerges as a result of circuit integration.
Monthly group meetings, close collaboration, and an active series of annual workshops
here at RIKEN by a distinguished international panel of scientists will guide
our quest. The first topic will be "Cellular
interactions in neuronal networks"
from April 3-5, 2001, featuring Y. Amitai, E. Buhl, E. Callaway, B. Connors, M.
Kano, Y. Kawaguchi, A. Konnerth, T. Manabe, I. Mody, S. Nelson, M. Scanziani,
R. Yuste, R. Wong. Wonユt you join us in Wako? (for further information: search14@brain.riken.go.jp)
<References>
1) Uchida, N., Takahashi, Y.K., Tanifuji, M., Mori, K. (2000) Odor maps in the
mammalian olfactory bulb: domain organization and odorant structural features.
Nat Neurosci. 3:1035-1043 2) Mori, K., Nagao, H., Yoshihara, Y. (1999) The olfactory
bulb: coding and processing of odor molecule information. Science 286:711-715
3) Yoshihara, Y., Mizuno, T., Nakahira, M., Kawasaki, M., Watanabe, Y., Kagamiyama,
H., Jishage, K., Ueda, O., Suzuki, H., Tabuchi, K., Sawamoto, K., Okano, H., Noda,
T., Mori, K. (1999) A genetic approach to visualization of multisynaptic neural
pathways using plant lectin transgene. Neuron 22:33-41 4) Hensch, T.K., Fagiolini,
M., Mataga, N., Stryker, M.P., Baekkeskov, S., Kash, S.F. (1998) Local GABA circuit
control of experience-dependent plasticity in developing visual cortex. Science
282:1504-1508 5) Fagiolini, M., Hensch, T.K. (2000) Inhibitory threshold for critical-period
activation in primary visual cortex. Nature 404:183-186 |
|
|
 |
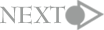 |
|
|