 |
Since our laboratory
started in September 2000, we have been working on several different projects
ranging from molecular to disease. But all are united in two key words: synaptic
plasticity and excitatory transmission. |
 |
 |
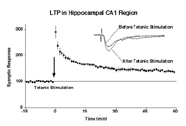
magnified
scene by clicking image
Fig.1 |
LTP
in hippocampal CA1 region. |
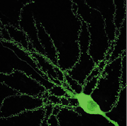
magnified
scene by clicking image
Fig.2 |
Dendritic
spines of CA1 pyramidal cells. Tiny puncta are spines. |
|
Molecular
Mechanism of Synaptic Plasticity
In the mammalian
hippocampus, a brief tetanic stimulation of input fibers leads to a long-term
potentiation (LTP) of excitatory synaptic transmission (Figure 1). LTP has been
widely studied as a cellular and synaptic model for learning and memory. The LTP
induction in hippocampal CA1 region requires post-synaptic NMDA receptor activation
and a resultant influx of Ca2+ ions. One consequence of this rise in postsynaptic
Ca2+ concentration is to trigger an increase of the transmission mediated by AMPA
receptors via an activation of various Ca2+-dependent protein kinases. However,
it has not been clear how this increase in transmission is attained. We suggested
that an activity-induced translocation of the AMPA receptor from the extrasynaptic
location to the postsynaptic site explains an increase in AMPA receptor transmission
after LTP induction. We demonstrated this by using GFP and electrophysiologically
tagged AMPA receptor molecules.
On the other hand, recent application of the yeast two-hybrid screening method
disclosed a complex network of protein-protein interaction underlying excitatory
synapse. This structural meshwork is not rigid but is dynamically regulated by
various stimuli. It is therefore highly likely that the dynamics of AMPA receptors
which themselves are embedded in this meshwork are regulated as a part of this
dynamism of the meshwork. The principal aim of our research project is to understand
the protein-protein interactions regulating AMPA receptor dynamics during LTP.
For this purpose, we employ multidisciplinary approaches including biochemical
isolation of glutamate receptor binding protein, construction of various mutants
of glutamate receptor itself and binding proteins, gene introduction into neurons,
and electrophysiological recording of those neurons.
Molecular
mechanism spine formation
Spines are major
site of excitatory synaptic transmission in hippocampal pyramidal cells (Figure
2). Their peculiar shape was first described as early as 19th century but the
knowledge how it is formed and how its number and shapes are regulated are still
limited. This is mainly due to a technical difficulty in accessing this structure.
However, recent advance in imaging technique, mainly two-photon microscope, enabled
direct visualization of spines in living neurons. The number and shape of the
spines is regulated during development as well as by synaptic activity. Hippocampal
CA1 pyramidal cells are virtually aspiny during the first two postnatal weeks
except for filopodial structures, which are much longer and thinner than typical
spine structure. After this period, typical spines with head and neck structure
start emerging. In addition, tetanic stimulation induces new spines. In order
to understand the mechanism involved in spine formation, we express various proteins
found in spines and to see if they can form spine by themselves or change the
morphology of spines. The spines are visualized with green fluorescent protein
(GFP) under two-photon microscope. When GFP is expressed in neuron, it fills cytosol
and thus depict the tiny protrusions of the cells including spines as if Golgi
staining. Electrophysiological recording and Ca2+ imaging will be combined with
GFP imaging to assess functional aspect of spines.
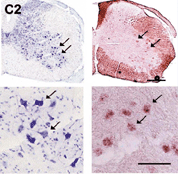
magnified
scene by clicking image
Fig.3 |
Generation
of knockout mice with tissue-specific deficiency using a Cre/LoxP recombination
system |
|
Structural
approach to understand postsynapse
To understand
the biophysical properties of synaptic protein from different aspect, we started
a project to reveal 3D structures of those proteins. We will express various postsynaptic
proteins in bacteria in large quantity, purify them, and make crystals. The resultant
protein crystal will be analyzed with X-ray defraction. This approach is auxiliary
the functional approach described above. For example, once functional unit of
a protein is identified the physiological experiment, the relevant fragment will
be used for crystallization trials. On the other hand, once we solved the structure
and find a motif which seems to have functional significance, we can make mutants
for further physiological analyses.
Role
of adult neurogenesis in learning and memory
One interesting feature of hippocampus is a continual regeneration of neurons
that takes place in adulthood. Placing an animal in a rich environment (for example,
provided with a place to hide etc.) increases neurogenesis. The neurogenesis also
takes place in olfactory bulb. However, there are no direct proof that the newly
generated neurons are actually involved in formation of neuronal circuit and hence
in a process of learning and memory. In order to test this, we are currently making
a transgenic animal model where neuronal stem cell can be removed by infusion
of cytotoxic substance. The resulting animal will be tested for memory paradigms
such as Morris water maze task or pregnancy block.
Application
of our knowledge to clinical medicine
We have recently identified a novel glutamate receptor subunit, NR3B, in the human
and mouse genomes. NR3B shows very restricted expression in somatic motoneurons
of the brainstem and spinal cord (Figure 3(. Due to this restricted expression,
we are interested if this gene is involved in any of motoneuron diseases exemplified
by amyotrophic lateral sclerosis (ALS).
ALS and motor neuron disease are neurological diseases that affect over 350,000
of the world's population, and kill over 100,000 every year. In the patients,
spinal motor neurons degenerate resulting in a loss of control of muscles and,
eventually, in a tragic death due to inability of respiration. Of the total ALS
cases, 90% is sporadic and 10% has family history. Some populations of familial
ALS cases have mutation in cytosolic Cu/Zn superoxide dismutase, an enzyme that
converts free radical - O2 to H2O2 and O2. However, it accounts for only 15-20%
of the familial cases and the cause the rest of familial and also most of sporadic
cases is not known. We are interested in the possibility that a mutation in NR3B
explains such familial ALS cases which cannot be attributed to SOD mutation and
now determining the sequence of DNA samples obtained from ALS patients. It will
help to understand the pathogenesis of ALS and motor neuron diseases, or even
neurodegenerative diseases in general. |
 |
 |
|
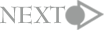 |
|
|