The development of functional brain imaging has led to significant progress in understanding the brain's function. The combination of such imaging techniques as fMRI and classical methods of cognitive science has given important correlates between particular behavioural reactions and parameters of activity in corresponding brain regions. To make further progress in brain research it is important to understand what happens at the level of cellular networks in these regions. Unfortunately, experimental techniques which can address questions at that level are still limited. Certain advances can be made using computational neuroscience. However, this approach is strictly dependent on available information about the mechanisms of brain cell communication and the anatomical structure of their connections.
Until recently, the networks of synaptically interconnected neurons were considered the main functional structures of the brain. This has historical reasons. More than a century ago, Ramón y Cajal described the main types of the neurons and their connections in the brain. This was the first significant advance made in cellular neuroscience with optical methods. Since that time, electrode based techniques have dominated the field. These techniques have demonstrated that neurons could generate electrical activity such as action potentials and postsynaptic potentials. Electrical and chemical synapses have been described. It was natural to view the brain as an electrical device and neurons as its elementary units integrating synaptic inputs. When, in the early seventies, synaptic plasticity (long-term potentiation and long-term depression) was described, it was proposed as the mechanism for learning and memory. Since then many laboratories have been trying to find experimental links between synaptic plasticity and memory.
Important changes in the view of how the brain works came with the idea of extrasynaptic interactions among brain cells. Around three decades ago, the concept of ‘volume transmission’ was formulated. It suggested that there is always some amount of agonist for neuronal receptors present in the extracellular space. This agonist acts on extrasynaptic receptors and modulates the activity of large groups of neurons. That was pioneering concept for its time, but unfortunately it nailed down that ‘extrasynaptic means modulatory’. Even now, quite a few neuroscientists still think that extrasynaptic action of transmitters is only modulation of synaptic networks. In the mid-nineties Dimitri Kullmann proposed neurotransmitter spillover as mechanism for intersynaptic crosstalk. He argued that glutamate released during synaptic events could escape synaptic cleft (spillover) and reach NMDA receptors at neighbouring synapses. This idea first associated extrasynaptic transmitter diffusion to signalling and suggested its transient nature.
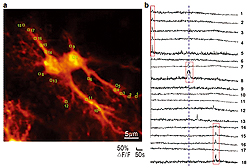
Fluorescence image (a) and recordings of Ca2+ transients (b) from a pair of hippocampal astrocytes containing calcium indicator (collaboration with Hirase Research Unit). Regions of interest (ROIs) marked with yellow boxes (a). Ca2+ transients recorded from ROI 1-18 before and after bath application of 200μM glutamate(the dashed blue line, b). The red boxes mark Ca2+ transients confined to the single compartment of the astrocytes (process or soma).
Figure by Mrs Pei‐Yu Shih
Another important discovery was that astrocytes, one type of glial cells, could release agonists of neuronal receptors. Sadly, since the first morphological studies these cells were in obscurity. Even the name ‘glia’ means glue. All non-neuronal cells are considered glue which fills the space between the neurons and electrically isolates them. In the era of electrode methods, it was reasonable to think that astrocytes are electrically inactive cells and therefore should not transfer any information. Neuron - astrocyte interactions were limited to nutrition of the neurons by the astrocytes. However, modern optical methods completely changed our view of astrocytes. Using fluorescent calcium imaging, including laser scanning microscopy, one can see calcium transients which appear in the astrocytes and their processes (Fig.1). This finding indicates that astrocytes are inactive electrically, but can generate a different kind of activity. The meaning of these calcium transients is not fully understood. Some scientists associate them with the extracellular release of transmitters, while others argue against that. Investigation of this intriguing issue is one of the directions of research in our laboratory.
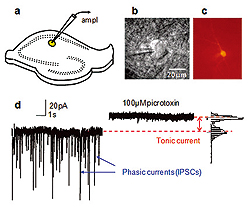
a. Schematics showing a hippocampal slice with patch clamp electrode on CA1 str.radiatum interneuron (yellow).
b. Infrared DIC image of interneurons with the tip of a patch pipette.
c. Interneurons filled with biocytin Alexa Fluor594 and visualized after the experiment to obtain detailed morphology of the cell.
d. Voltage clamp recording in presence of NMDA and AMPA receptor antagonists. Spontaneous IPSCs originating from spontaneous presynaptic GABA release and spontaneous presynaptic action potentials are blocked by 100μM picrotoxin. In addition, picrotoxin produces a shift in holding current by blocking tonic current mediated by extrasynaptic GABAA receptors.
Figure by Dr. Inseon Song
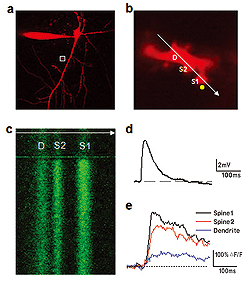
a. Neuron filled with calcium indicator Fluo-5F (300μM) and morpho logical tracer Alexa Fluor 594 (50μM). Only Alexa Fluor 594 channel showing neuron morphology is presented.
b. Enlarged image of a branch of apical dendrite (boxed region in a). It also indicates the position of line-scan (white arrow) and point of two-photon uncaging (yellow circle). D is the dendrite, S1 and S2 are spines. Simultaneous imaging and uncaging is performed using a two-scanner imaging system Olympus FV1000.
c. Line-scan image of Fluo-5F fluorescence across two spines and parent dendrite. Increase in fluorescence is evoked by uncaging of MNI-glutamate.
Depolarizing potential induced in soma of the neuron by glutamate uncaging (d) and corresponding increases in calcium in dendrite and 2 spines (e).
Figure by Mr Yu‐Wei Wu
In contrast to the original concept of modulatory volume transmission, recent findings suggest that the effects of extracellular transmitters can be fast and transient. For instance, when glutamate escapes from a synaptic cleft or is released by an astrocyte, it reaches a relatively high concentration in narrow extracellular cleft. Then it diffuses from the place of release, and transporters take it back into neurons and astrocytes. As result, only high affinity receptors in proximity of the release point can be activated. In principle, these receptors can be both synaptic and extrasynaptic. However, synapses are often isolated by astrocytic processes containing high transporter density. This protective cap limits the access of extrasynaptic agonists to the synaptic receptors. Freely accessible extrasynaptic receptors are easier targets for extrasynaptic transmitters.
Understanding of the conditions for non-synaptic receptor activation is another research direction in our laboratory. Currently we are investigating extrasynaptic ionotropic GABAA and NMDA receptors. These receptors are activated by GABA, the main inhibitory neurotransmitter, and glutamate, the main excitatory neurotransmitter, respectively.
Extrasynaptic GABAA receptors can detect ambient GABA concentrations. Compared to their synaptic cousins these receptors have higher affinity to GABA and slower kinetics of desensitization. This allows them to mediate persistent tonic current (Fig.2). Such tonic current has been found in the neurons of many brain regions. The presence and density of extrasynaptic GABAA receptors, the proximity of GABA release sources, and density of GABA transporters near the cell determine the magnitude of the tonic current. As result, some neurons express more tonic current than others. Such cell type specificity has important network implications. When ambient GABA increases during certain types of behaviour, it produces larger tonic current in particular neurons within the local network than in others, and this determines specific information processing within such network.
Neuronal extrasynaptic NMDA receptors do not mediate persistent current at physiological conditions. Even when glutamate is available in the extrasynaptic space; the channel of these receptors is blocked by Mg2+ ions. This voltage dependent block prevents receptor activation. It can be removed by neuronal depolarization such as EPSP or action potential. Because NMDA receptors have high calcium permeability, we employ two-photon fluorescent calcium imaging to study their activation in the spines and dendrites of hippocampal neurons (Fig.3).
Another mystery is related to NMDA receptors found in astrocytes. These receptors can also detect glutamate concentrations, but astrocytes do not fire action potentials or generate EPSPs to relieve a Mg2+ block. Therefore, these receptors should not be blocked by Mg2+ and can generate current each time they are exposed to glutamate. What is the function of this current in electrically inactive cells? We have some ideas. If you want to know, please visit our lab.